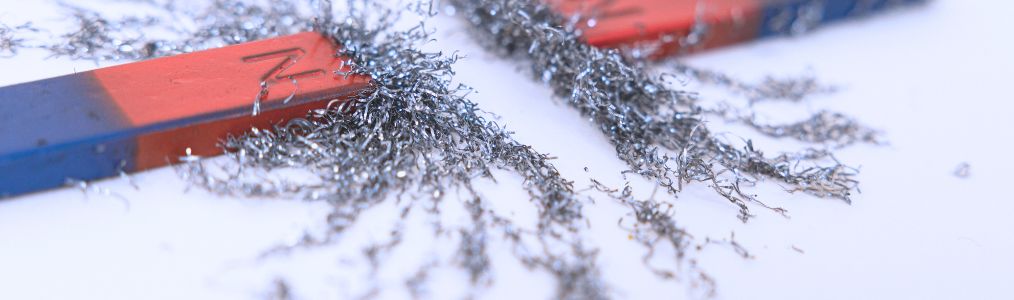
“Superparamagnetism enable our nanomaterials to create previously unimaginable possibilities,” says Dr. Jérémy Paris, CEO of SON.
What is Superparamagnetism?
Louis Néel discovered superparamagnetism in 1949 during a study on fired clay and lava (“Theory of Magnetic Drag of Fine-Grain Ferromagnetics with Applications to Fired Clays,” Louis Néel). The work of this Nobel Prize-winning physicist helped to understand the phenomenon and to use SPION (SuperParamagnetic Iron Oxide, or iron oxide nanoparticles) in many applications.
Superparamagnetism: The Magnetism of the Nano World
Magnetism is a more complex phenomenon than it might seem. It exists in various forms, and to grasp the nuances, we’ll start with a few definitions.
- Magnetism
Magnetism refers to a set of physical phenomena where objects exert forces of attraction or repulsion on other materials. It includes behaviors like ferromagnetism, ferrimagnetism, paramagnetism, and superparamagnetism.
- Magnetic moment
A magnetic moment is a vector quantity representing the magnetic force and orientation of magnetization produced by an object. In the presence of a magnetic field, the magnetic moment aligns and orients accordingly.
- Ferromagnetic material
A ferromagnetic material is divided into multiple magnetic domains (called Weiss domains) separated by boundaries (called Bloch walls). In each domain, all magnetic moments align in the same direction. However, without an external field, the orientations of the magnetic moments across different domains vary. If no magnetic field has influenced the material, the total magnetization is zero. When a magnetic field is applied, all domains align in the same direction, creating strong magnetization. Once the field is removed, the domains partially realign randomly, leaving behind some residual magnetization.
- Ferrimagnetism
Ferrimagnetism is a variation of ferromagnetism. Both are magnetic behaviors that exhibit Weiss domains. In ferrimagnetism, within each domain, the magnetic moments are aligned with each other but oriented in opposite directions, with greater intensity in one direction than in the other.
Thus, in the presence of an external magnetic field, the total magnetization produced by a ferrimagnetic material is lower than that of a ferromagnetic material. When the external field is removed, ferrimagnetic compounds also exhibit residual magnetization.
- Paramagnetism
Paramagnetism refers to the magnetization of a material when exposed to a magnetic field. Without the field, the magnetic moments are randomly oriented, and the material lacks magnetization. When exposed to a magnetic field, the moments align, causing the material to become magnetized, albeit weakly compared to ferromagnetic materials.
- Superparamagnetism
Superparamagnetism refers to the behavior of nanoscale ferrimagnetic and ferromagnetic materials in the presence of a magnetic field.
Each nanoparticle has a larger overall magnetic moment compared to paramagnetism, allowing for stronger magnetization in response to an external field.
Like paramagnetic materials, their magnetic properties are only activated only when a magnetic field is nearby. Superparamagnetism is therefore similar to paramagnetism, but with more intense magnetization under the influence of an external magnetic field.
The key takeaway is that paramagnetism and superparamagnetism primarily differ in the size of their magnetic moments.
How Do Magnetism, Paramagnetism, and Superparamagnetism Work?
The distinction may seem complex, but it’s simpler than it appears. Here’s how it works.
When a ferromagnetic or ferrimagnetic material is heated above its Curie temperature, it loses its permanent magnetic properties and becomes paramagnetic. The Curie temperature is the point where thermal agitation causes the aligned magnetic moments to disalign. Each material has a specific Curie temperature, for example, 358°C for nickel, 768°C for iron, and 1,127°C for cobalt.
However, to transition from ferromagnetism to superparamagnetism, heating is unnecessary. By reducing a material to the nanoscale, Bloch walls (the transition zone between magnetic domains) disappear, as maintaining them at this scale would require too much energy. Only a single domain remains, and these nanoparticles are called monodomains.
The magnetic moments of the nanoparticles cancel each other out and only align under the influence of an external magnetic field.
What Are the Practical Applications of These Properties?
Superparamagnetic properties of iron oxides, cobalt ferrites, or nickel ferrites offer considerable interest in industry and medicine.
Revolutionizing Catalysis: The Impact of Superparamagnetism
Catalysis is fundamental in industries like chemical, petrochemical, pharmaceutical, and energy, as it accelerates chemical reactions and improves product selectivity. SON has developed nanocatalysts utilizing the superparamagnetic properties of SPION. By modifying the surface of iron oxide nanoparticles, SON has grafted catalytic metals such as platinum or ruthenium. These nanocatalysts are magnetic, making them easy to recover and reuse without additional purification.
Using an external magnetic field (such as a magnet), the SON catalysts can be recovered, simplifying post-reaction product separation. Gone are the days of complex, costly, and time-consuming filtration and centrifugation techniques! These superparamagnetic catalysts can be reused for up to 10 cycles without losing efficiency.
An Innovative Solution for Pollutant Cleanup
The magnetic properties of these nanomaterials have inspired a new way to purify industrial wastewater or water. Superparamagnetic nanoparticles, such as iron oxides, can be functionalized to bind to specific pollutants, known as SON’s nanoscavengers. These materials capture metals, which can then be recovered using a magnetic field.
Nanomedicine: A Promising Advancement in Diagnosis and Therapy
In medicine, superparamagnetic nanoparticles (like iron oxides) are used in magnetic resonance imaging (MRI). They serve as nanocarriers to identify, target, and attack tumors at an early stage, such as glioblastomas or melanomas, which SON is currently researching. Their magnetic properties and nanoscale size enable them to cross certain natural barriers in the human body, such as the blood-brain barrier. Additionally, through magnetic hyperthermia, these superparamagnetic nanomaterials can be used to kill infectious organisms once targeted.
The Challenges of Superparamagnetism
To maximize the capabilities of superparamagnetic nanoparticles, several conditions must be met. The size of the nanoparticles is critical, which is why SON specializes in developing highly characterized, reproducible nanomaterials. It’s essential to consult experts when harnessing superparamagnetic properties to ensure nanoparticle quality and detailed analytical certifications.
SON, a producer and designer of nanomaterials, is one such expert, available to assist with your projects. Please contact us for more information.